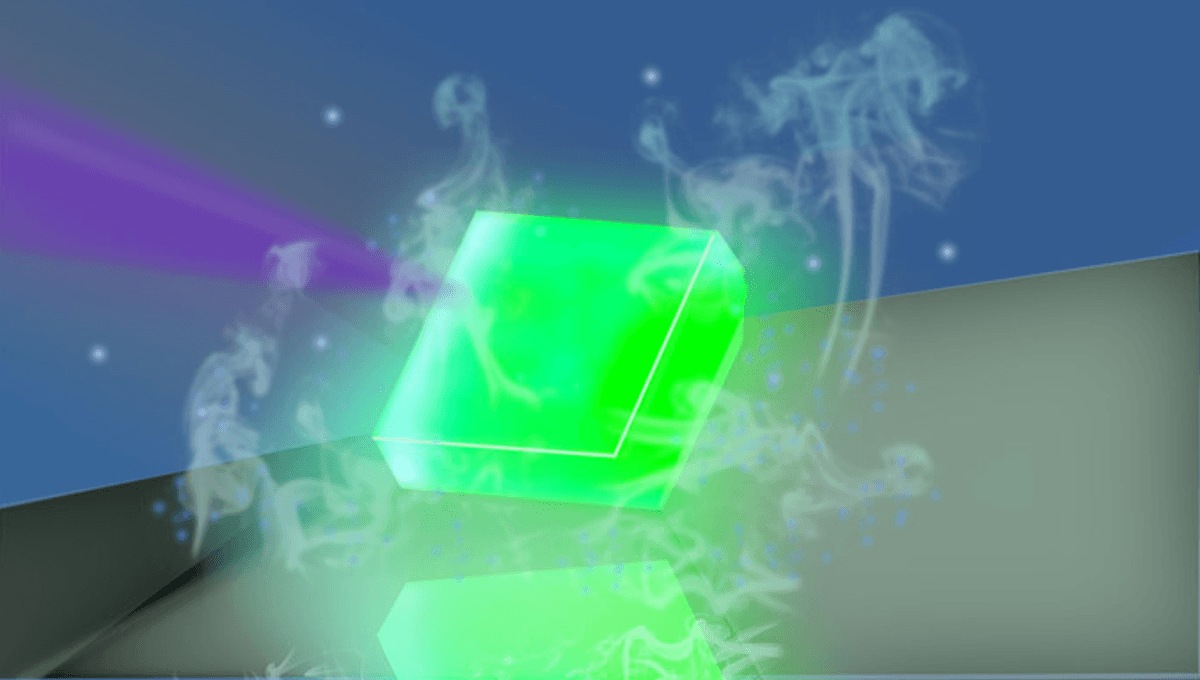
Quantum dots can cool themselves by turning low energy light into higher-energy photons, which are radiated away. The idea, which runs against classical physics, might avoid the need to expend energy to actively reduce temperatures, although the economics are doubtful, even in a world struggling to keep its cool.
Every now and then, residents of warm climates are bombarded with online adverts for portable cooling devices that sound attractive, until you realize they break the second law of thermodynamics. Since most physicists consider this to be the law in all of physics least likely to ever be proven wrong, a fan costing under $100 probably won’t violate it for you, so the possibility of a scam should be considered.
At first sight, new work led by Professor Yasuhiro Yamada of Chiba University might appear similar, but it’s not. The physics it violates, while good enough for introductory courses and most circumstances, has already been shown to have exceptions. Yamada and colleagues have taken advantage of these to cool quantum dots and the crystals around them by more than anticipated.
The team used the phenomenon of anti-Stokes photoluminescence, where photons get absorbed and then released at higher energies. In normal photoluminescence, as seen in a surprising number of animals, a material absorbs light and releases it at longer wavelengths, which carry less energy.
Ordinary photoluminescence is relatively easy to explain. The absorbing molecule relaxes a little and sheds some energy before reradiating. It’s a great deal harder to add extra energy to a system.
When light falls on certain semiconductor, electrons and positively charged “holes”, collectively known as excitons, are released. This production forms the basis of photovoltaic cells.
However, in many circumstances, instead of producing electricity, the electrons and holes recombine and release light again. Unfortunately, when the production of excitons becomes too high for the space, the excitons undergo Auger recombination. The resulting photons have such short wavelengths they are heat, rather than visible light, warming the semiconductor up.
It might seem we are doomed to have to cool overheated semiconductors using traditional methods, such as blowing air over them. However, Yamada and coauthors were aware that in anti-Stokes photoluminescence, the semiconductor’s crystal lattice’s vibrations impart energy to the excitons, causing the release of photons with shorter wavelengths than those that made them. In the process, heat is removed.
The difficulty here is that anti-Stokes emission efficiency is never 100 percent. While some higher energy photons carry away heat from the system, other excitons produce more heat under Auger recombination. The challenge is to get the efficiency high enough that the cooling effect outweighs the warming one.
“Efforts to achieve optical cooling in semiconductors have encountered several difficulties, primarily due to challenges in reaching nearly 100% emission efficiency, and true cooling has been elusive. Though quantum dots are promising for their high emission efficiency, they are notoriously unstable, and exposure to air and continued illumination degrade their emission efficiency. Thus, we focused on a stable structure known as ‘dots-in-crystals,’ which may overcome these limitations,” Yamada said in a statement.
The team made their crystals of of perovskite, the wonder-structure just starting to transform the solar power industry, as well as offering a variety of other applications.
The cooling effect is inherently limited, because at low intensity the light can’t achieve much, and as the intensity rises efficiency declines. However, Yamaba’s team showed that with the right surrounding crystal and careful selection of light intensity, they can cool a room temperature array of quantum dots by 9 °C (16 °F). That doesn’t take the dot-in-crystals down to even the temperature inside a household fridge. However, in the context of previous estimates of a 1 °C (1.8 °F) ceiling, it’s impressive.
Applications, if any, are likely to be restricted to highly specialized circumstances. You’ll probably never cool your room by turning the light on, but you’d still have a better chance than with those science-denying fans.
Light has been used for cooling before, and indeed is the standard way to get very small numbers of atoms close to absolute zero. However, that is a very different method, using the pressure of lasers to stop atoms’ random oscillation, and is not thought suited to scaling up.
The study is published in NanoLetters.
Source Link: Shining A Light On A Superconductor Can Cool It Down